Mechanic
Wednesday, 19 February 2025
Tuesday, 18 February 2025
How 3D CAD Software is Revolutionizing Packaging Design and Engineering
A mechanical device in mechanical engineering refers to any machine, tool, or apparatus designed to perform a specific function by utilizing mechanical principles such as motion, force, and energy conversion. These devices range from simple mechanisms like levers and pulleys to complex machinery such as engines, turbines, and robotic systems. The fundamental purpose of mechanical devices is to facilitate work, enhance efficiency, and improve precision in various engineering applications.
One of the most common types of mechanical devices is the gear system, which consists of interlocking toothed wheels that transmit motion and force between rotating shafts. Gears are widely used in automobiles, industrial machinery, and even in household appliances to control speed and torque. Another essential mechanical device is the hydraulic system, which operates based on Pascal’s Law, stating that pressure applied to a confined fluid is transmitted equally in all directions. Hydraulic systems power heavy machinery such as excavators, cranes, and aircraft landing gear by converting fluid pressure into mechanical work.
In the automotive industry, internal combustion engines (ICEs) serve as a critical mechanical device, converting chemical energy from fuel into mechanical energy through controlled explosions within cylinders. These engines power vehicles, generators, and industrial equipment, making them indispensable in modern transportation and energy sectors. However, with advancements in technology, electric motors are increasingly replacing ICEs in many applications due to their efficiency and environmental benefits.
Another significant category of mechanical devices includes robotic arms, which are programmable machines capable of performing precise and repetitive tasks in manufacturing, medical surgeries, and space exploration. These robotic systems utilize actuators, sensors, and controllers to mimic human hand movements, improving productivity and accuracy in industrial processes.
Mechanical devices also play a crucial role in thermal power plants, where steam turbines convert thermal energy from steam into mechanical energy to generate electricity. These turbines are widely used in power generation plants and rely on principles of thermodynamics to maximize efficiency. Similarly, refrigeration and air conditioning systems employ compressors and heat exchangers to regulate temperature by transferring heat from one space to another.
The development of additive manufacturing, commonly known as 3D printing, has introduced a new dimension to mechanical devices by enabling rapid prototyping and production of intricate components. This technology uses layer-by-layer material deposition to create complex parts, revolutionizing industries such as aerospace, medical implants, and custom manufacturing.
In conclusion, mechanical devices are the backbone of engineering, influencing nearly every aspect of modern life. From basic tools to advanced machinery, these devices enhance efficiency, safety, and functionality in industries ranging from transportation and energy to healthcare and automation. As technology advances, mechanical devices will continue to evolve, integrating smart systems, AI, and automation to further improve their performance and capabilities.
Social Media Links:
Monday, 17 February 2025
Advancements in Rapid Prototyping Technologies
With these breakthroughs, the journey from concept to reality is faster and more flexible than ever.
The Evolution of Rapid Prototyping
3D Printing: A Revolution in Creation
Speed and Innovation
READ MORE: How Design for Excellence (DfX) Intersects with Rapid Prototyping
Material Versatility
Cost Efficiency
Laser Cutting: Precision Redefined
Exploring Other Notable Rapid Prototyping Methods
Saturday, 15 February 2025
3D-Printing Flexible Devices without Mechanical Joints
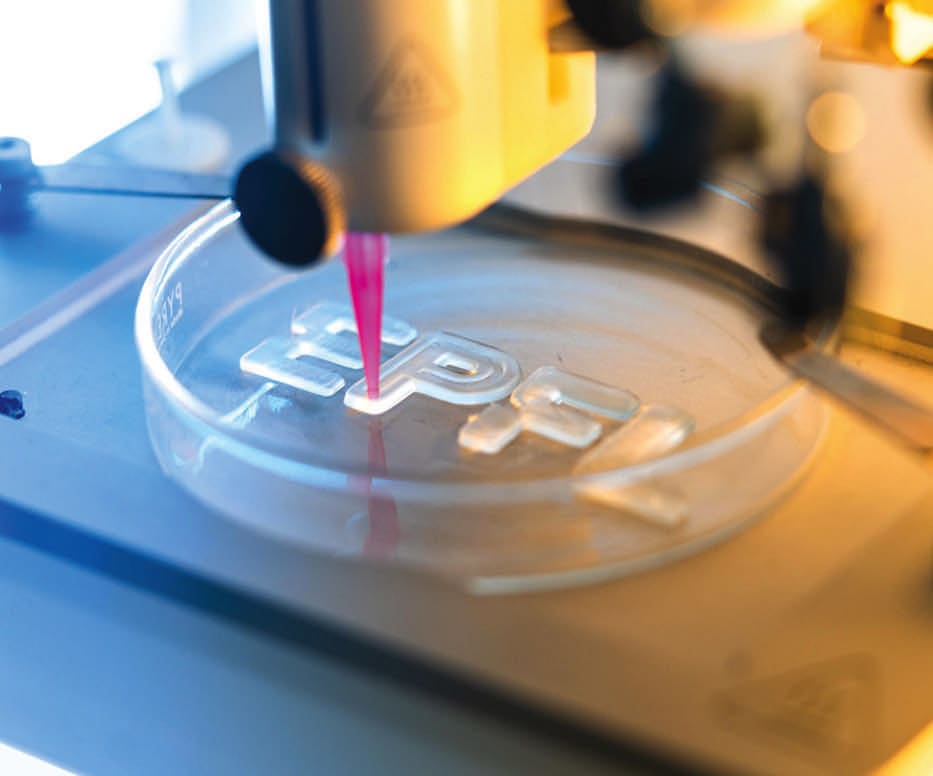
For more information, contact Celia Luterbacher at celia.
Friday, 14 February 2025
Manufacturing Processes with AI-Powered Visual Inspection Configurable without programming skills, the MELSOFT VIXIO AI-powered visual inspection software is revolutionising quality assurance across industries.
How does it specifically work?
- Learning phase: The software learns what a "good" product looks like by reviewing many examples of good and bad items, similar to studying for a test.
- Image processing: After learning, it analyses product images in real-time using algorithms to compare them against its training data.
- Decision making: Based on its analysis, the software gives products a "thumbs up" if they meet standards or a "thumbs down" if not, ensuring only quality products are sent out.
- Feedback loop: The software improves over time; if it makes mistakes, engineers can update its learning data to enhance its accuracy in recognising products.
Thursday, 13 February 2025
Emerging 2D Materials for Future Electronics
Image Credit: Golubovy/Shutterstock.com
Why are 2D Electronic Materials Needed?
What are the Applications of 2D Electronics?
What 2D Materials Will Be Used in Future Electronics?
Emerging System-on-a-Chip Trends to Watch Out For
References and Further Reading
Leveraging Predictive AI in Telecommunications with RAN Intelligent Controller (RIC)
In the dynamic landscape of telecommunications, the RAN Intelligent Controller (RIC) has emerged as a transformative technology. The trans...
-
CNC MACHINING CNC 101: The term CNC stands for 'computer numerical control', and the CNC machining definition is that it is a su...
-
CAD for rapid prototyping and 3D printing #news : CAD for rapid prototyping and 3D printing #visit : https://cad-conferences.sciencefather...
-
Parametric and feature-based modeling techniques in CAD #news : Parametric and feature-based modeling techniques in CAD #visit : https://c...